Biological and Soft Matter
Principal Investigators
- Dr Sebastian Ahnert
- Dr John Biggins
- Dr Rosana Collepardo
- Prof Ben Simons
- Dr Anton Souslov
- Dr Sarah Teichmann
This programme of activities is supported by regular seminars and workshops through the Edwards Centre for Soft Matter, the post-doc led Theory of Living Matter Group, and a University-wide network associated with the Cambridge Centre for Physical Biology.
Some of the greatest advances in biological and biomedical sciences have been underpinned by technological innovations developed in the physical sciences. From biological structure determination, electron microscopy, NMR, mass spectrometry, to recent developments in next-generation sequencing, single molecule fluorescence microscopy, and single-cell multi-omics, physical scientists have created the enabling technologies that have driven progress in the field.
Through these developments, our understanding of subcellular processes, through the interactions of genes and gene products have evolved. Yet, despite these advances, the translation of this knowledge into higher-level biological function remains surprisingly limited. With increasing access to quantitative measures of biological activity, these challenges open new horizons for the development of statistical and computational approaches to understand the mechanisms that regulate biological function at the cellular, tissue and organismal scale. To contribute to this expanding field of Physical Biology, the TCM group and its close affiliates in Cambridge are developing new analytical and computational tools to address biologically-inspired phenomena across a range of length scales.
Computational Molecular Biology
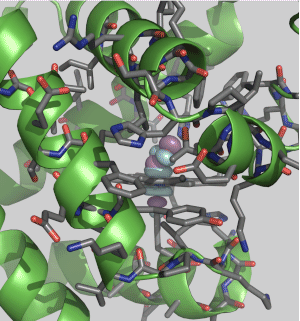
Through the development of 'linear scaling algorithms' allied with an increase in computational processing power, the functional interactions and chemistry of complex biological molecules are beginning to become accessible to density functional theory approaches. By targeting paradigmatic problems in the biochemistry of biomolecular compounds, including enzyme chemistry, drug design, and photosynthesis, we are helping to lay the foundations for a new field of Computational Molecular Biology, which will lead to new conceptual insights into the molecular regulation of basic biological processes.
Theory of living matter
As complex multicomponent systems operating far from equilibrium, the unpredictable character of biological systems make them an unlikely arena for theoretical physicists. Yet, in common with driven inanimate systems, the non-equilibrium dynamics of statistical ensembles of equivalent biological entities (such as biomolecular assemblies of genes and gene products, cells, bacterial colonies, swarms, etc.) can lead to the emergence of new 'collective phenomena', defined by few composite variables and macroscopic length scales. Elucidating the existence and the physical basis of such collective phenomena calls for the development of computational and analytical approaches that draw upon concepts from mathematics and theoretical physics. By collaborating closely with experimental biology labs, researchers in TCM and their affiliates are addressing core areas of molecular, stem cell and developmental biology. Current projects include functional genomics, evolutionary biology, protein assembly, tissue morphogenesis, genetic lineage tracing, stem cell biology, and the emergence of diseased states.
Soft Matter
The category of 'soft' encompasses many different types of matter, including liquid crystals, polymers, gels, elastomers, colloids and foams, united by having large responses to modest stresses. In TCM, our work on soft matter focuses on two categories of mechanically soft solids. We work on elastomers that contain liquid crystal order, a new phase of soft matter in which large shape changes can be produced by heat and light, or imposed to produce dramatic colour changes, and the mechanical response of simple soft solids, such as gels elastomers and biological tissues, to large applied strains.
Liquid crystal elastomers
Liquid Crystal Elastomers (LCE) are rubbery networks composed of long, crosslinked polymer chains that are also liquid crystalline - nematic, cholesteric or smectic: they are solid rubbers containing liquid crystalline order. The liquid crystalline order strongly influences the conformations of the polymer strands and hence the macroscopic shape of the solid. In the simple case of a nematic LCE, if the sample is heated, the nematic order is destroyed, and the sample shortens. If it is cooled again, the order reappears and the sample stretches along the nematic alignment direction. These shape changes can be very large, up to 500%. Liquid crystal order can also be manipulated inside the rubber. For example it can be rotated by electric or magnetic fields, again leading to large shape changes, or induced to rotate by applying mechanical stretch. Many other exotic phenomena arise with other liquid crystal phases. Cholesteric rubbers have a photonic stop band, giving them striking colours, which change when stretched and the ability to lase. SmA rubbers have exhibit perfect 2-D elasticity. SmC* rubbers have large-strain piezoelectricity. Photo-elastomers loose order when illuminated, giving large fast controllable shape changes with possible uses in actuators.
Simple soft solids
Soft solids, such as gels, elastomers, and biological tissues, can undergo reversible shape changes of several hundred percent. The elastic theory of soft solids must account for these geometrically large shape changes, and this results in new shape-changing elastic instabilities that are universal to all sufficiently soft solids. In TCM we seek to discover and explain these shape changing elastic instabilities. We also investigate whether these shape-changing instabilities are harnessed by evolution to sculpt the shape of biological organs during development. For example, do the folds on the surface of the human brain arise simply because the cerebral cortex grows faster than the interior and mechanically buckles?
Mechanical metamaterials
To develop novel mechanical properties, materials can be structured on a scale much larger than the molecular or microscopic. This structure can be used to design novel mechanical properties in what are called mechanical metamaterials. In these materials, sound, vibrations, and elastic moduli can be controlled in a more precise way compared to a simple, atomic or molecular, material. One useful design principle for new metamaterials is topology, which deals with properties that remain invariant under continuous change. A broad challenge for mechanical metamaterials is to design exotic properties inaccessible in the simple materials available to us.
Active matter
Active matter refers to materials in which the constituents use an energy source to move themselves. Active matter is broadly present in the natural world, from bacterial colonies to tissues. Recently, synthetic active matter has been designed and controlled in the lab, from collections of polymers pushed around by molecular motors to colloids that catalise reactions on their surface. The principles of active matter can be used to understand biological function, and to design new synthetic materials and devices.
Current research topics in the group include:
- active matter (Souslov)
- soft aerosols and microdroplets (Souslov)
- complex biological networks (Ahnert, Teichmann)
- computational molecular biology (Collepardo, Payne)
- liquid crystal elastomers (Biggins)
- protein assembly and dynamics (Ahnert, Collepardo, Teichmann)
- human cell atlas (Teichmann)
- stem cell biology and cancer (Simons)
- tissue morphogenesis (Biggins, Simons)