Christopher Parmee
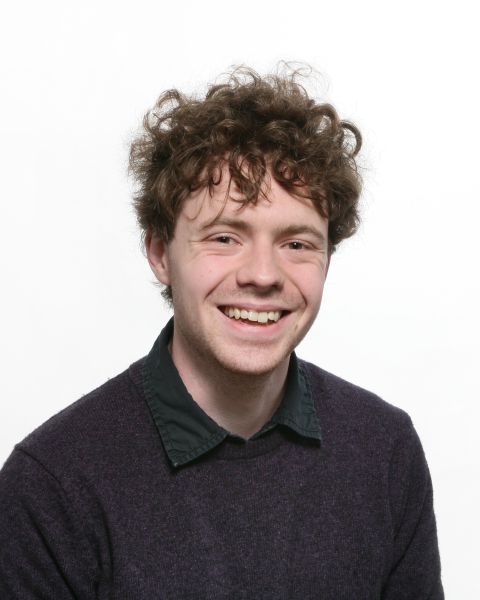
Christopher Parmee
Member of Robinson College
PhD student in Prof Cooper's group
Office: 511 Mott Bld
Phone: +44(0)1223 3 37261
Email: cp487 @ cam.ac.uk
TCM Group, Cavendish Laboratory
19 JJ Thomson Avenue,
Cambridge, CB3 0HE UK.
Research
My research involves theoretical studies of emergent phenomena in ultra cold atomic gases due to the collective many-body physics of strongly interacting quantum systems.
My main focus is on the role of dipole interactions in cold atomic ensembles, Rydberg gases and polar molecules with only two accessible energy levels. These systems behave as effective spin models, where the dipole coupling results in long-range interactions. These long-range interactions can significantly alter the collective behaviour of the ensemble when driving the atoms or molecules with an external field and this changes how light propagates and scatters through the ensemble.
Of particular interest is the role of these effects in open quantum systems, where excitations can decay into an environment. Dipole interactions in these systems not only lead to long-range interactions but also intriguing dissipative properties, such as the emergence of nonlocal dissipation. Nonlocal dissipation results in the formation of super-radiant and sub-radiant collective decay modes, where excitations in the system either decay much faster or slower than a single excitation would on its own. The interplay of long-range interactions and nonlocal dissipation leads to novel non-equilibrium dynamics and long-time steady state phases.
In Plain English
Collective phenomena is any occurence where particles behave together and is a key area of study in condensed matter physics, leading to interesting effects such as Bose Einstein condensation, magnetism and superconductivity. When we consider how light interacts with a material, we usually treat the atoms as independent oscillators, where the electron and atom behave as if joined by a spring and the light causes the spring to move back and forth. This model is relatively successful at describing most light-matter interactions we see in everyday life. However, if we make the temperature low enough, we have to take into account quantum effects and interactions between the atoms. This can give rise to collective behaviour which changes how light behaves when it enters a material and means we have to formulate new models to describe these processes.
Featured Publications
- Decay rates and energies of free magnons and bound states in dissipative XXZ chains arXiv:1812.07893
- Phases of driven two-level systems with nonlocal dissipation Phys. Rev. A 97 (2018) , arXiv:1712.04296
- Stable collective dynamics of two-level systems coupled by dipole interactions Phys. Rev. A 95 (2017) , arXiv:1612.02595